1.0 Introduction.
Starch modification is an evolving field which explores different techniques that can be used to improve important properties of starch. The composition of starch (mainly amylose/amylopectin ratio) from different sources varies. However, due to its structure, a wide range of modifications are possible, leading to the enhancement of desired properties [1]. Different starch sources have been explored in the development of packaging films, but cassava starch is of huge industrial interest as its source (cassava) is highly abundant, especially in tropical countries.
Cassava has low lipid content, low retrogradation tendency and high gel stability [2]. For development of packaging films, starch has been modified through various chemical methods including oxidation, hydrolysis, etherification and esterification; all targeted at addressing the challenges associated with starch-based films which are mainly mechanical property deficiencies and water sensitivity [3]. One modification method of interest for enhancing film properties is cross-linking. The concept of crosslinking involves the introduction of inter and intra molecular bridges or linkages between the glucose chains in starch, using a crosslinker. The crosslinks developed during these reactions create a rigid network within the starch structure which could address the water sensitivity and mechanical property deficiencies associated with most starch-based films [4]. Crosslinking reactions, however, are very sensitive and their efficiency is dependent on factors such as: the type of crosslinker, concentration, pH and the starch composition (amylose/amylopectin ratio, chain length, branching framework) [5].
Epichlorohydrin, glutaraldehyde, phosphoryl chloride, sodium trimetaphosphate (STMP) sodium tripolyphosphates (STPP) are crosslinkers that have been well studied and effectively used to modify starch, however, the current emphasis on cost effectiveness and environmental friendliness of any industrial process has led to research into other bio-based crosslinkers [4,5]. Some studies have also highlighted the toxicity and regulatory concerns surrounding the use of the above chemical crosslinkers [6]. Bio-based crosslinking involves the use of natural or non-toxic reagents for crosslinking reaction and which generates non-hazardous by-products in the process. Bio-based crosslinkers such as citric acid [7], oxidized sucrose [8], malonic acid [9] and tartaric acid [10] have been mentioned in literature.
While there are a good number of reports of citric acid for crosslinking of different starches, oxidized sucrose crosslinking of starch has hardly been reported. To fully explore the prospective application of both crosslinkers in modifying starch for film applications, it is important to evaluate their individual and combined effects on the key properties of cassava starch that influence the mechanical property and stability of starch-based films [7,8]. This study explores the potential of oxidized sucrose and citric acid for the modification of cassava starch to be utilized in packaging films, by understanding their effects on some physicochemical and functional properties. The properties studied include amylose content, degree of substitution (DS), degree of crosslinking (DC), swelling power, solubility and paste clarity. The study also draws relationships between the properties of starch and their influence on film formation. The results obtained then suggest the suitability of each crosslinker for the modification of cassava starch in development of packaging films.
2.0 Materials and Methods
2.1 Starch Extraction
The Methods reported by Fadeyibi et al. [11] were used to extract starch. Cassava tubers were obtained from the local market in Minna-Niger State, Nigeria; they were peeled, diced, soaked in clean water for 20 hrs and washed afterwards. They were ground to paste and subsequently sieved through a sieve cloth. The filtrate was then allowed to stand for 12 hrs, the settled starch was then collected and re-suspended in distilled water for another 12 hrs. After this period, the supernatant was discarded while the clean starch was collected and dried at 50 oC in an oven.
2.2 Starch cross-linking
2.2.1 Oxidized sucrose crosslinking
This involved a two-step procedure: first, the preparation of oxidized sucrose before crosslinking with starch using methods described by Wang et al. [12]. Sodium periodate (12.90 g) and 6 g of starch were dissolved in distilled water (200ml volume) after which the solution was then stirred at room temperature for 26 hours. Afterwards, 7g of barium chloride was added and the solution further stirred for 60minutes, maintaining the temperature at 5oC. The solution was then filtered, and the filtrate (oxidized sucrose) stored at 5oC. The different concentrations of oxidized sucrose (0.1%, 0.5%, 1%, 2.5%, 5%, 7.5% and 10%) in 100ml were prepared, 100g of starch dissolved and subsequently stirred for 6 hours. On completion of the reaction the starch was then washed three times with distilled water, completely oven dried at 40°C and stored in sealable bags for characterization.
2.2.2 Citric acid crosslinking
This was carried out as described by Kim et al. [13] with some slight modifications. The different concentrations (0.1%, 0.5%, 1%, 2.5%, 5%, 7.5% and 10%) of citric acid in distilled water were prepared and the pH of the solution was adjusted to 3.5 using NaOH. Starch (100g) was added to the citric acid solution and the solution stirred for 18 hr. After the reaction the starch was washed, and dried
2.2.3 Dual modification
Starch was first crosslinked with oxidized sucrose as described in 2.2.1 and then subjected to citric acid treatment described in 2.2.2.
2.3 Starch characterization
2.3.1 Determination of amylose.
The methods described by Taghvaei-Ganjali et al. [14] was used to determine the amylose content with some modifications. Test tubes were set with 4ml of Dimethyl-sulphoxide (DMSO) and 16mg of each starch sample was dissolved in them. All the test tubes were pre-heated for 15 minutes in a water bath set at 80oC and then left to stand for 45 minutes at room temperature. One ml of each starch solution was then added to 50mls of already prepared iodine reagent (iodine and potassium iodide) and made up to 50mls with distilled water. The absorbance of each sample at 600nm was measured using a spectrophotometer. The amylose content of each sample was then extrapolated from a standard amylose curve (developed using pure amylose).
2.3.2. Determination of degree of substitution (DS)
The simple method described by Hustiany [15] was used to determine the DS of the crosslinked starches. 2g of each starch sample was dissolved in 50ml distilled water, 25mls of 0.5M sodium hydroxide added and stirred for 30minutes. The excess sodium hydroxide was then titrated against 0.1 M HCl. The DS was calculated using the formula DS = 162 M (S – B) / 1000 W
Where M is the Molarity of HCl (mol /litre), W is the weight of sample (g), B is the volume of 0.1 M HCl in the blank (ml) and S the volume of 0.1 M HCl in the sample (ml)
2.3.3 Determination of the degree of crosslinking (DC)
Measurement of the DC was done using peak viscosity values obtained through a Rapid Visco Analyser (RVA) as described by Shah et al. [5]. Three grams of each crosslinked starch was dissolved in 30mls of distilled water and then heated from 50°C to 95 °C (at about 12 °C/min). The starch solution was then held at 95 °C for 2 minutes, cooled to 50 °C (at the rate of 12 °C/min) and finally kept at 50 °C for 2 min. The peak viscosity values were then obtained and the degree of cross-linking determined using the relationship below:
DC = (A-B) / A×100
A = peak viscosity of the native starch
B = peak viscosity of the crosslinked starch.
2.3.4 Determination of swelling power and solubility
The methods described by Kusumayanti et al.[16] was used to determine the swelling power of crosslinked starches. Dry centrifuge tubes were pre-weighed with 0.2g of each starch sample and 20ml of distilled water added. The tubes were then heated at varying temperatures of 30°C, 50°C, 70°C and 90°C for 30mins with periodic manual stirring. After the heating process, the tubes were then centrifuged at 1500rpm for 30 mins, the supernatant water decanted and the sedimented starch weighed. The swelling power (SP) was then calculated.
SP (g/g) = Weight of Starch paste
Initial weight of starch
The sedimented starch was then dried completely at 100oC, the solubility was then calculated:
Solubility (%) = Weight of dried Starch residue x 100
Initial weight of starch
2.3.5 Paste Clarity and stability
Each starch sample (0.1g) and 10mls volume of distilled water were placed in dry screw cap tubes and mixed thoroughly. The tubes were then heated in a water bath at 90°C for 30minutes after which they were allowed to cool to room temperature. The clarity of the solution was determined using a spectrophotometer as a function of the transmittance at 650nm against a water blank as described by Sit et al. [17]. The stability of the starch samples was measured by storing at 4℃ in a refrigerator, the absorbance was then measured after every 24 hours for five days.
2.3.6 Fourier transform Infrared (FTIR) spectroscopy.
Starch samples were analysed by FTIR (FTIR-8400S Fourier Infrared Spectrophotometer) using potassium bromide pellets. Samples were scanned 10 times from 600 to 4000cm -1 at a resolution of 2cm-1. The spectra results were then subjected for ATR correction utilising the OMNIC software of the FTIR equipment [12].
2.3.7 Thermal Analysis
The thermal behavior of starch samples was studied using the thermogravimetric analyzer (Perkin Elma STA 6000). Starch samples (0.6 g) were dissolved in 18mg of distilled water, placed in the pans and left to stand for 70minutes. The pans were heated from 25°C to 900°C at a rate of 10°C per minute and the thermographs obtained [12].
2.3.8 Scanning Electron Microscopy
The morphology of the starch granules before and after crosslinking were studied using scanning electron microscope (model: PRO:X: 80007334 Phenom World, MVE01570775). A thin layer of each starch granule was mounted on the aluminum specimen holder by a double-sided tape. The samples were coated with platinum and examined under the microscope at an accelerating voltage of 15 kV with magnification of 1000X [13].
2.3.9 X-ray Diffraction
The diffraction patterns of native and crosslinked starches were observed using X-ray diffractometer (Thermoscientific model: ARL XTRA, 197492086). A current of 40 mA and voltage of 40 kV was used with Cu Kα radiation (λ=1.54Å) and the diffraction angle (2θ) ranging from 5° to 40° [12].
2.4 Statistical Analysis
The statistical analysis was conducted in triplicates using Statistical Package for Social Science (SPSS) version 21, One Way Analysis of Variance (ANOVA) and Duncan’s Multiple Range Test (DMRT) were used for comparison. Data values were expressed as means ± SEM and considered significant at P < 0.05.
3.0 Results and Discussion
3.1 Determination of crosslinker concentration through effect on the Amylose content and DS.
The amylose content and DS were used to determine the most effective concentration of both crosslinkers to be used in this modification. In Figure 1, it was observed that the highest amylose content for OS-starch (20.78%) was achieved at 1% concentration, while that of CA-starch (19.4%) was achieved at 5%; Beyond these concentrations, the values of amylose content began to decline. In Figure 2, highest values for DS were also observed at 1% and 5% concentrations of OS-starch and CA-starch respectively. All values of DS observed in this study (0.182 – 0.401) were within intermediate range.
The concentration of the crosslinker is an important factor during modification. At extremely low concentrations, the crosslinks produced might be insufficient to effect significant property improvement; also at high concentrations, the excessive crosslinks developed could limit the mobility of starch molecules and hamper property improvement [18]; hence the importance of determining the concentration that has the highest effect on the physicochemical properties of starch which is indicative of film strength. Some studies recommend as low as 0.1% crosslinker concentration while most studies report less than 10%concentration, depending on the crosslinker, the starch source and intended application [5]. For packaging film applications, an increase in amylose content is desired after modification as higher amylose content has been associated with improved film properties in most studies [19].
A report on cassava starch showed that during film preparation, the linear chains of amylose interact better to form hydrogen bonds with the plasticizer when compared to amylopectin which has a higher level of entanglement as a result of its branched configuration [20]. Higher amylose content is believed to enable uniform water evaporation during the drying phase which leads to a more stable polymer matrix and consequently better mechanical properties [21]. Molecular studies have also shown that during crosslinking, most linkages occur between amylopectin molecules, while only a few crosslinks occur between amylose and amylopectin molecules [22]. There are also suggestions that crosslinks created could lead to the un-branching of a few amylopectin molecules hence leading to an increase in amylose content [23]. The DS indicates the average number of hydroxyl groups in the glucose units in starch that have been replaced during any modification and has a maximum value of 3. Similar to amylose, most studies show that a higher DS after modification corresponds to better film properties [24]. Increase in DS also indicates that less hydroxyl groups of glucose units are available for interaction between water. This lowered water interaction leads to lower starch mobility and better interaction with plasticizer during film formation; hence increase in DS should lead to increase in film tensile strength [25]. Some studies however report that a very high DS (above 2.4) tends to restrict film biodegradability [1]. Trela et al. [26] also explains that a high DS could instigate surface changes in starch granules as well as loss of crystalline structure hence making them highly unsuitable for film formation.
The optimal concentration of oxidized sucrose and citric acid agree with most studies which record between 0.1 to 5%. Shandu et al. [27] varied the concentration of epichlorohydrin between 0.1 and 1% on crosslinking sorghum starch and the results showed that different concentrations of the crosslinker had optimal effect on different physicochemical properties studied. The increase in amylose content observed in this study agrees with reports by Sudhakara and Parimalavalli [28] where the amylose content of corn starch was higher after crosslinking while another study reported a higher amylose content of yam starch after crosslinking with STMP [29]. The results for the DS in this study are higher than the values reported using other crosslinkers; a degree of substutitution of 0.14 was reported for STPP/stearic acid crosslinked cassava starch [15] while another study showed a DS of 0.12 after corn starch was crosslinked with gluteraldehyde [30]. Higher values for DS (1.41) have also been reported after crosslinking rice starch with STMP/STPP [31]. The results in this study therefore suggest that based on amylose content and DS, 1% and 5% are the most effective concentrations of oxidized sucrose and citric acid respectively.

Figure 1: Effect of oxidized sucrose and citric acid concentration on amylose content. Each dot represents the average of three replicates.
3.2 Effect of dual modification on amylose content, DS and DC
A dual modification (combination of both crosslinkers) was done using 1% oxidized sucrose and 5% citric acid while the amylose content, DS and DC were observed. From Table 1 it was observed that the amylose content of the OS-CA starch was significantly (p<0.05) lower than that of OS-starch but significantly (p<0.05) higher than that of CA-starch. No significant (p<0.05) difference was observed between the DS and the DC of OS-starch and OS-CA starch.
Dual modification attempts to combine the potential property improvement from the single modifications and has been reported to have varying effects on different properties of starch depending on the source of starch as well as the sequence and conditions of reactions involved [32]. While the amylose content and DS are key parameters considered in any starch modification, the DC is specific to crosslinking modifications and describes the relative measure of the number of covalent bonds produced during the crosslinking reaction or the extent to which successful crosslinks have been created. Increase in the DC has been suggested to lead to improvement in film mechanical properties as higher number crosslinks ensures less starch mobility and a more rigid conformation [33]. A particular study showed that higher DC corresponded to an increase in tensile strength when the effect of different crosslinkers on the mechanical properties of rice starch film was observed [33].

Figure 2: Effect of oxidized sucrose and citric acid concentration on DS. Each dot represents the average of three replicates.
A few studies highlight the effect of dual modification on amylose content and DC. A lower amylose content was observed after dual modification of yam starch when compared to crosslinking with STMP alone [29]. Huaxi et al. [34] reported a DC of 20% in dual crosslinked potato starch which is lower than values observed in this present work. Higher values for DC (47.34% – 58.28%) were reported after dual modification of lotus (Nelumbo nucifera) rhizome starch [35] while the dual modification of banana starch in another study had DC between 21.99% and 46.74% [36].
Overall, dual modification did not significantly improve the amylose content, DS and DC of cassava starch beyond the values observed after oxidized sucrose crosslinking, indicating that film properties also might not be further enhanced by this combined treatment.
Table 1. Summary of Amylose content, degree of substitution (DS) and degree of crosslinking (DC)
Modified Starch (Concentration) | Amylose Content | Degree of Substitution | Degree of Crosslinking |
OS (1%) | 20.78 ± 0.06d | 0.406 ± 0.01b | 27.4± 0.53b |
CA (5%) | 19.4 ± 0.31b | 0.388 ± 0.01a | 20.8± 0.46a |
OS – CA (1% / 5%) | 20.01 ± 0.42c | 0.401 ± 0.16b | 28.3 ± 0.73b |
Native Starch | 18.11 ± 0.14a |
Data are presented as Mean± Standard Deviation of triplicate determinations. Values with different superscript alphabets across the row are significantly different at p<0.05.
3.3 Effect of crosslinking on swelling power and solubility.
In Figure 3, all crosslinked starches showed similar swelling patterns when temperature was increased. The swelling power of OS-starch began to decline slightly after 50 oC, while the decline was observed after 70 oC for both CA-starch and OS-CA starches. The swelling power of the native starch however consistently increased with increase in temperature. Overall, it was observed that at 90°C there was a significant difference between the swelling power of native starch (30.83g/g) and all the crosslinked starches (5.62 – 12.01 g/g), with OS-starch having the lowest swelling power in all the temperatures studied.
In Figure 4, the solubility of all starches increased with increase in temperature, however the crosslinked starches had lower solubility values at all temperatures observed. This indicates that although heating generally increases the solubility of starch, crosslinked starches were less soluble at higher temperatures. It was also observed in this study that at the extreme temperatures (30oC and 90oC), the solubility of OS-CA starch was lower than that of both OS-starch and CA-starch while at 50◦C and 70◦C the solubility was higher than the both starches.
Swelling power and solubility are important properties of modified starches as they highlight the interaction between glucose chains in the amorphous and crystalline regions of starch in solution. Their patterns are highly influenced by the nature of crosslinking, DC, starch granule size and temperature [12]. At temperatures close to gelatinization temperature, the crosslinks further strengthens the structure of starch and prevents leaching, hence at this point the resistance to swelling is much higher [37]. The formation of C-O-C crosslinking bonds between the aldehyde groups of oxidized sucrose and the hydroxyl groups of starch could be vital in inhibition of water intrusion [12]. Increase in crosslink density has also been suggested to restrict the disintegration of starch granules when close to gelatinisation [38] while other reports show that crosslinking induces a more rigid configuration, thereby restricting the penetration of water molecules and subsequently limits the swelling in starches [5].
Similar patterns in swelling power were observed in STMP/STPP crosslinked banana starches which showed a reduction in swelling power when temperatures were increased from 60 oC to 90 oC [39], while another report showed that oxidized sucrose crosslinked corn starch had much lower swelling power than the native starch [8]. Mirmoghtadaie et al. [40] reported a significantly lower swelling power in oat starch crosslinked with phosphorous oxychloride and this was attributed to the restricted mobility of starch chains in the amorphous regions. Some studies suggest the ability of amylose conformation to inhibit starch swelling while amylopectin promotes swelling [3]. The increase in amylose content of crosslinked starches in this study may therefore have supported the lowered swelling power. For development of biodegradable films, restricted swelling enhances the uniformity during drying of starch solution which potentially improves the strength of the films. Lower solubility and swelling power have also been reported after crosslinking maize starch [41] and kudzu starch [42].
The lowered swelling power and solubility in this study indicates that crosslinking reduced the hydrophilic property of cassava starch and therefore suggests that films produced would have higher water resistance in addition to the enhanced mechanical properties which are important in packaging film applications
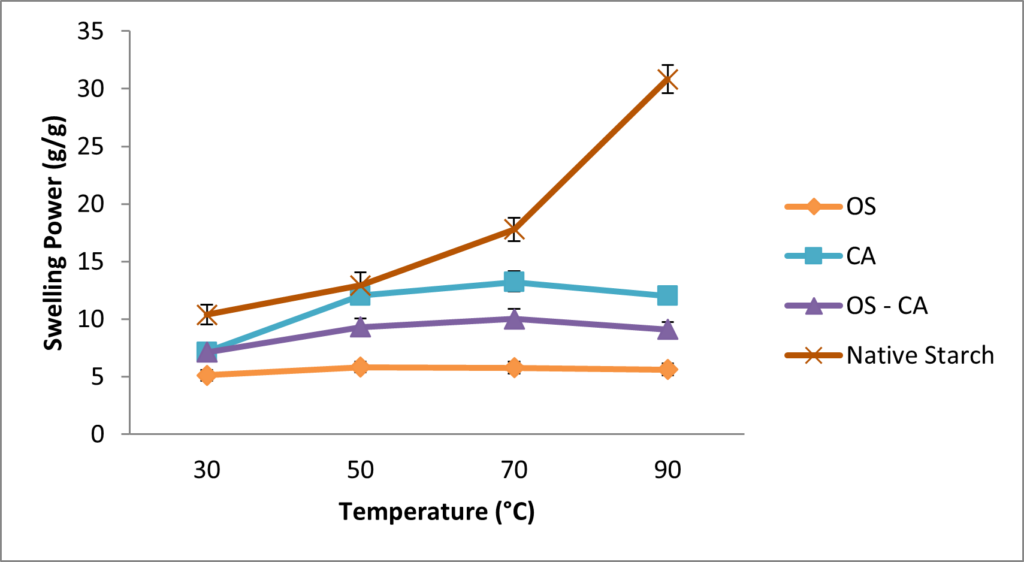
Figure 3: Effect of temperature on the swelling power of native and crosslinked cassava starch. Each dot represents the average of three replicates.

Figure 4: Effect of temperature on the solubility of native and crosslinked cassava starch. Each dot represents the average of three replicates.
3.4 Effects of crosslinking on paste clarity and stability
In Figure 5, the paste clarity of all starches were observed as well as the stability over 5 days. The results indicate that the paste clarity of crosslinked starches were much lower than the native starches; also, CA-starch had a higher paste clarity than OS-starch and the OS-CA starches while the latter had a stable absorbance throughout the five day period.
Paste clarity index describes light transmission through starch paste and gives an insight into the potential transparency of films produced from starch. The compact conformation of starch granules as a result of the crosslinks developed and substituted hydroxyl groups will definitely reduce the transmission of light across the starch molecules leading to a lowering of paste clarity [3]. The results in this study agree with reports of lower paste clarity observed in epichlorohydrin crosslinked rice starch [37], STMP/STPP crosslinked corn starch [38], epichlorohydrin crosslinked cassava starch [43] and STMP crosslinked cassava starch [44]. The results therefore suggest that based on higher paste clarity, the CA-starch could produce more transparent films when compared to films from OS and OS-CA starch.

Figure 5: Paste clarity of native and crosslinked cassava starch. Each dot represents the average of three replicates.
3.5 Fourier-transform infrared (FT-IR) spectroscopy
The combined infra-red spectra profile of both native and crosslinked starches are described in Figure 6. The results of all crosslinked starches showed a significantly striking double peak at 2924 cm-1 and 2862 cm-1 which correspond to the stretching vibration of carbon hydrogen (C-H) bonds. Bands at 1742 cm -1 in OS-CA-starch and 1748 cm-1 in both OS-starch and CA-starch are associated with bending vibrations of the hydroxyl groups, while a hydroxyl group deformation is observed at 1450cm-1. Peaks at 1087cm-1 however indicate the streching vibration of C–O bond. The presence of peaks between 3000 cm -1 and 3500 cm -1 is an indication of available OH groups that have not been subject to crosslinking or substitution reactions. Similar peaks were reported by Utomo et al. [45] between 3000 and 3600 cm-1 which denoted available OH groups and also between 2800 and 3000 corresponding to vibration of CH bonds when citric acid was used to crosslink tapioca starch. The intensity of the peaks at 1134cm-1 can be seen to be much lower in the OS-starch and CA-starch and disappeared in the dual crosslinked starch indicating the continuous disruption of crystallinity after treatment with both crosslinkers. The peaks at 1011 depicts the amorphous structure of starch. The functional groups observed in this analysis affirms that crosslinking with oxidized sucrose, citric acid and dual modification were successful in modifying the structure of cassava starch.
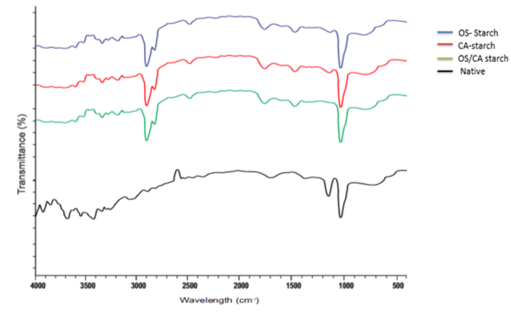
Figure 6: Infra-red spectra of native and crosslinked starches
3.6 Scanning electron microscopy (SEM)
The SEM micrographs of native starch (NS) and crosslinked starches as shown in Figure 7 indicate only slight changes in the morphology of crosslinked starches. The presence of cracks on the surfaces of all the crosslinked starches could be observed as the surfaces appear rougher than the native starches. The native starch appears to have areas where the starch granules clump, as this could be attributed to the post drying conformation after starch extraction. The OS-starch and OS-CA starches appear to be more tightly packed and uniformly distributed as compared to the CA-starch and native starch. The presence of higher number of crosslinks (as observed from the higher DC and DS) could contribute to the tightly packed but uniform conformation. The SEM results in this study are similar to other reports including the cracks on the surface lining of citric acid crosslinked tapioca starch [45] and the irregular shaped morphology of corn starch granules after crosslinking [28] while another study observed that cyperus starches appeared more uniform after crosslinking [46]. The micrographs show that the oxidized sucrose had higher effect on the morphology of cassava starch which further suggests that it might be better suitable for modifying starch for film applications.
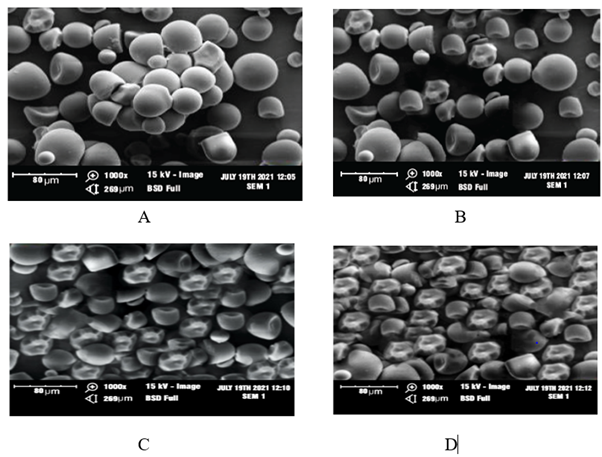
Figure 7: SEM images of native crosslinked starches. A, B, C and D representing native starch, CA-starch, OS-starch, and OS-CA starch respectively.
3.6 X-ray diffraction (XRD)
Results from the XRD analysis are shown in Figure 8 and it can be observed that the native starch showed peaks at 15.2°, 17.1°, 18.3° and 23.2° which corresponds to A type crystalline pattern. No distinct change in the crystalline patterns of CA-starch was observed, however the OS-starch and OS-CA starch showed lower intensity of peaks at 15.2°, 17.1° and 18.3° as well as a complete disappearance of the peak at 23.2° suggesting a slight disruption of the crystalline structure of starch. Similar diffraction patterns were seen in citric acid crosslinked rice starch, however in this study a total disappearance of peaks was observed at the high concentration of citric acid (30%) [13]. Some other reports show no pronounced changes in x-ray diffraction patterns after the crosslinking of corn starch [38,47]. The emergence of a V type crystalline pattern after crosslinking corn starch with oxidized sucrose has also been reported [8]. This result indicates that although slight changes were observed, all the crosslinking modifications did not totally disrupt the crystalline structure of cassava starch.
3.7 Thermal analysis
The result in Figure 9 describes the effect of crosslinking on the thermal behaviour of starch. The TGA graphs of crosslinked and native starches (Figure 9A) showed two stages of weight loss; between 80°C and 120°C and between 260°C to 400°C. For the native starch, the residual weight percentage of the native starch was 13.23% while that of the crosslinked starches was 19.59%, 18.74%, and 19.68% for OS-starch, CA-starch and OS-CA starch. The DTG plots in Figure 9B showed the (peak decomposition temperature (Tp) for native starch was 308.12° while Tp values of 326.52°, 324.93° and 326.78° were recorded for oxidized sucrose, citric acid and dual crosslinked starches respectively.
The initial stage of degradation occurred as a result of initial evaporation of water upon heating while the second stage could be attributed to the onset of thermal degradation due to the molecular dehydration reactions within starch molecules. Beyond second stage, heating results in carbonisation and subsequent ash formation. Different studies highlight the improvement in thermal stability after crosslinking. This can be attributed to the formation of crosslinking networks within starch, creating more resistance to deformation by heat. The higher DC reported for OS-starch and OS-CA starches (as seen in Table 1) could be responsible for the significantly higher residual weight observed in both starches after heating when compared to the native starch.
The thermal stability of starch generally depends on the amylose/amylopectin conformation, molecular weight and modification conditions. After modification, increase in DS has been reported to lead to an increase in thermal stability; this trend was attributed to the lowered amount of hydroxyl groups remaining after modification [48]. Increase in thermal stability of starch has also been associated with lower retrogradation tendency during film formation [49, 18]. This could suggest that higher thermal stability of starch could lead to more uniform films with improved properties. Studies on rice starch films highlighted the effect of starch retrogradation on the overall properties of the films, however, more detailed study was suggested so as to fully understand the mechanisms involved [50]. There are also reports of films with higher decomposition temperature after crosslinking, and this was attributed to compact internal structure of films as well as stronger bonds formed between starch and the crosslinkers as compared to the bonds between starch and the plasticizer [50].
A residual weight of 20% was reported for corn starch crosslinked with oxidized sucrose [8] while another report showed an increase in peak temperature after the crosslinking of sago starch with citric acid [51]. The results therefore suggests that crosslinking conferred significant improvement in the thermal properties of the cassava starch and subsequently, films produced from the crosslinked starch could have enhanced thermal stability.
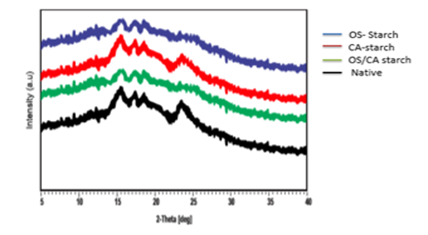
Figure 8: X-ray diffraction patterns of native and crosslinked starches

Figure 9: TG curves (A) and DTG curves (B) of native starch cross-linked starch of native starch and cross-linked starch
4.0 Conclusion
This present study showed that oxidized sucrose crosslinking, citric acid crosslinking and dual modification led to an increase in amylose content, a lowering of the swelling power, solubility and paste clarity as well as improvement in the thermal stability of cassava starch. Oxidized sucrose however, had a higher effect on most of the properties studied hence can be suggested to more suitable for modifying cassava starch intended to be utilized in the development of packaging films.
Ethics approval and consent to participate: Not applicable
Consent for publication: Not applicable
Competing interests: The authors declared no conflict of interest exist
Funding: This research received no external funding
Authors’ contributions: The work was conducted in collaboration of all authors. All authors read and approved the final version of the manuscript.
References
1. Chiu, C.; Solarek, D. Modification of Starches. Chapter 17. Starch 2009. 629-655.
2. Breuninger, W. F.; Piyachomkwan, K.; Sriroth, K. Tapioca/cassava starch: production and use. In Starch 2009, pp. 541-568. Academic Press.
3. Alcázar-Alay, S.C.; Meireles, M.A. Physicochemical properties, modifications and applications of starches from different botanical sources. Food Science and Technology International 2015, 35, 215-236.
4. Wittaya, T. Rice starch-based biodegradable films: properties enhancement. Structure and function of food engineering 2012, 5, 103-134.
5. Shah, N.; Mewada, R.K.; Mehta, T.A. Crosslinking of starch and its effect on viscosity behaviour. Reviews in Chemical Engineering 2016, 32, 265 – 270.
6. Hirsch, J.B.; Kokini, J.L. Understanding the Mechanism of Cross‐Linking Agents (POCl3, STMP, and EPI) Through Swelling Behavior and Pasting Properties of Cross‐Linked Waxy Maize Starches. Cereal Chemistry 2002, 79, 102-107.
7. Owi, W.T.; Lin, O.H.; Sam, S.T.; Villagracia, A.R.; Santos, G.N. Tapioca Starch Based Green Nanocomposites with Environmental Friendly Cross-linker. Chemical engineering transactions 2017, 56, 463-468.
8. Xu, H.; Canisag, H.; Mu, B.; Yang, Y. Robust and Flexible Films from 100% Starch Cross-Linked by Biobased Disaccharide Derivative. ACS Sustainable Chemistry & Engineering 2015, 3, 2631-2639.
9. Dastidar, T.G. ; Netravali, A.N. ‘Green’crosslinking of native starches with malonic acid and their properties. Carbohydr. Polym.2012, 90, 1620–1628.
10. Mendoza, A. Tartaric Acid Cross-Linking of Starch: Effect of Reaction Conditions on the Maximum Tensile Strength of Cast Plastic Films. J. Stud. Sci. Technol. 2015, 8, 41–47.
11. Fadeyibi, A.; Osunde, Z.D.; Egwim, E.C.; Idah, P.A. Performance evaluation of cassava starch-zinc nanocomposite film for tomatoes packaging. Journal of Agricultural Engineering 2017, 48, 137-146.
12. Wang, P.; Sheng, F.; Tang, S.W.; ud-Din, Z.; Chen, L.; Nawaz, A.; Hu, C.; Xiong, H. Synthesis and Characterization of Corn Starch Crosslinked with Oxidized Sucrose. Starch/Stärke 2019, 71, 1–8.
13. Kim, J.Y.; Lee, Y.; Chang, Y.H. Structure and digestibility properties of resistant rice starch cross-linked with citric acid. International Journal of Food Properties 2017, 20, 2166 – 2177.
14. Taghvaei-Ganjali, S.; Motiei, F.; Shakeri, I.; Abbasian, A. Effect of amylose/amylopectin ratio on the physic-mechanical properties of rubber compounds filled by starch. Journal of Applied Researches IN Chemistry (JARC) 2010, 4, 53-60.
15. Hustiany, R. Characteristics of Crosslink Acylation Tapioca Substituted Nagara Beans (Vigna Unguiculata spp.Cylindrica) Flour. Agro industrial Journal 2014, 3, 125-132.
16. Kusumayanti, H.; Handayani, N.A.; Santosa, H. Swelling Power and Water Solubility of Cassava and Sweet Potatoes Flour. Procedia environmental sciences 2015, 23, 164-167.
17. Sit, N.; Misra, S.; Deka, S.C. Characterization of Physicochemical, Functional, Textural and Color Properties of Starches from Two Different Varieties of Taro and Their Comparison to Potato and Rice Starches. Food Science and Technology Research 2014, 20, 357-365.
18. Reddy, N.; Yang, Y. Citric acid cross-linking of starch films. Food Chemistry. 2010,118, 702-711.
19. Liu, Z.; Han, J. H. Film-forming characteristics of starches. Journal of Food Science 2005, 70, E31–E36.
20. Alves, D. V.; Mali, S.; Beleia, A.; Grossmann, E. M. Effect of glycerol and amylose enrichment on cassava starch film properties. Journal of Food Engineering 2007, 78 (3), 941-946.
21. Wittaya, T. Rice Starch-Based Biodegradable Films: Properties Enhancement. In A. A. Eissa (Ed.), Structure and Function of Food Engineering 2012,103-134.
22. Jane, J.; Ao, Z.; Duvick, S.A., Wiklund, M.; Yoo, S.; Wong, K.; Gardner, C.A. Structures of Amylopectin and Starch Granules: How Are They Synthesized? Journal of applied glycoscience 2003, 50, 167-172.
23. Delval, F.; Crini, G.; Bertini, S.; Morin-Crini, N.; Badot, P.M.; Vebrel, J.; Torri, G. Characterization of crosslinked starch materials with spectroscopic techniques. Journal of Applied Polymer Science 2004, 93, 2650-2663.
24. Garg, S.; Jana, A.K. Effect of propylation of starch with different degrees of substitution on the properties and characteristics of starch-low density polyethylene blend films. J. Appl. Polym. Sci. 2011, 122, 2197-2208.
25. Schmidt, V.C.; Blanco-pascual, N.; Tribuzi, G.; Laurindo, J.B. Effect of the degree of acetylation, plasticizer concentration and relative humidity on cassava starch films properties. Food Science and Technology 2019, 39, 491–499.
26. Trela, V.D.; Ramallo, A.L.; Albani, O.A. Synthesis and Characterization of Acetylated Cassava Starch with Different Degrees of Substitution. Brazilian Archives of Biology and Technology 2020, 63, e20180292.
27. Sandhu, K.S.; Siroha, A.K.; Punia, S.; Sangwan, L.; Nehra, M.; Purewal, S.S. Effect of degree of cross linking on physicochemical, rheological and morphological properties of sorghum starch. Carbohydr. Polym. Techno. Appl. 2021, 1, 100073.
28. SudhakaraRao, J.; Parimalavalli, R. Effect of cross-linking on structural, chemical and functional properties of corn starch. International Journal of Food Sciences and Nutrition 2019, 4, 135-140.
29. Sukhija, S.; Singh, S.; Riar, C.S. Effect of Oxidation, Cross-Linking and Dual Modification on Physicochemical, Crystallinity, Morphological, Pasting and Thermal Characteristics of Elephant Foot Yam (Amorphophallus paeoniifolius) Starch. Food Hydrocolloids 2016, 55, 56–64.
30. Gonenc, I.M.; Us, F. Effect of Glutaraldehyde Crosslinking on Degree of Substitution, Thermal, Structural, and Physicochemical Properties of Corn Starch. Starch – Stärke 2018, 71, 3-4.
31. Shen, Y.; Zhang, N.; Xu, Y.; Huang, J.; Yuan, M.; Wu, D.; Shu, X. Physicochemical properties of hydroxypropylated and cross-linked rice starches differential in amylose content. International journal of biological macromolecules 2019, 128, 775-781.
32. Ashogbon A. O. Dual modification of various starches: Synthesis, properties and applications. Food chemistry 2021, 342, 128325.
33. Detduangchan, N.; Sridach, W.; Wittaya, T. Enhancement of the properties of biodegradable rice starch films by using chemical crosslinking agents. International Food Research Journal 2014, 21, 1225-1235.
34. Huaxi, X.; Qinlu, L.; Qiang, L.; Yue, W.; Wei, T.; Wei, W.; Xiang-jin, F. Physicochemical properties of chemically modified starches from different botanical origin. Scientific Research and Essays 2011, 6, 4517-4525.
35. Sukhija, S.; Singh, S.; Riar, C.S. Molecular characteristics of oxidized and cross-linked lotus (Nelumbonucifera) rhizome starch, International Journal of Food Properties 2017, 20, S1065-S1081.
36. Babu, A. S.; Naik, G. N. M.; Jiya, J.; Aboobacker, A. B.; Akshaya E.; Mohan, R. J. A comparative study on dual modification of banana (Musa paradisiaca) starch by microwave irradiation and cross-linking. Journal of Food Measurement and Characterization 2018, 12, 2209-2217.
37. Xiao, H.; Lin, Q.; Liu, G.; Yu, F. A Comparative Study of the Characteristics of Cross-Linked, Oxidized and Dual-Modified Rice Starches. Molecules, 2012, 17, 10946 – 10957.
38. Koo, S.H.; Lee, K.Y.; Lee, H.G. Effect of cross-linking on the physicochemical and physiological properties of corn starch. Food Hydrocolloids, 2010, 24, 619-625.
39. Carmona‐Garcia, R.; Aguirre‐Cruz, A.; Yee‐Madeira, H.; Bello‐Pérez, L.A. Dual modification of Banana Starch: Partial Characterization. Starch/Stärke 2009, 61, 656-664.
40. Mirmoghtadaie, L.; Kadivar, M.; Shahedi, M.R. Effects of cross-linking and acetylation on oat starch properties. Food Chemistry 2009, 116, 709-713.
41. Liu, J.; Wang, B.; Lin, L.; Zhang, J.; Liu, W.; Xie, J.; Ding, Y. Functional, physicochemical properties and structure of cross-linked oxidized maize starch. Food Hydrocolloids 2014, 36, 45-52.
42. Chen, B.; Dang, L.; Zhang, X.; Fang, W.; Hou, M.; Liu, T.; Wang, Z. Physicochemical properties and micro-structural characteristics in starch from kudzu root as affected by cross-linking. Food chemistry 2017, 219, 93-101.
43. Jyothi, A. N.; Moorthy, S. N.; Rajasekharan, K. N. Effect of Cross-linking with Epichlorohydrin on the Properties of Cassava (Manihot esculenta Crantz) Starch. Starch – Stärke 2006, 58, 292–299.
44. Heebthong, K.; Ruttarattanamongkol, K. Physicochemical properties of cross‐linked cassava starch prepared using a pilot‐scale reactive twin‐screw extrusion process (REX). Starch-starke, 2016, 68, 528-540.
45. Utomo, P.P.; Nizardo, N.M.; Saepudin, E. Crosslink modification of tapioca starch with citric acid as a functional food. Proceedings of the 5th International Symposium on current progress in Mathematics and Sciences (ISCPMS2019). 2020 AIP Conference Proceedings 2242, 1. http://dx.doi.org/10.1063/5.0010364
46. Olayemi, B.; Isimi, C. Y.; Ekere, K.; Johnson Isaac, A.; Okoh, J. E.; Emeje, M. Green Preparation of CA starch for Improvement of Physicochemical Properties of Cyperus Starch. Turkish journal of pharmaceutical sciences 2021, 18, 34–43.
47. Shi, M.; Gu, F.; Wu, J.R.;Yu, S.; Gao, Q. Preparation, physicochemical properties, and in vitro digestibility of cross-linked resistant starch from pea starch. Starch-starke, 2013, 65, 947-953.
48. Liu, X.; Wang, Y.; Yu, L.; Tong, Z.; Chen, L.; Liu, H.; Li, X. Thermal degradation and stability of starch under different processing conditions. Starch – Starke. 2013, 65, 48–60.
49. Marta, H.; Cahyana, Y.; Bintang, S.; Pramafisi, G.; Djali, M. Physicochemical and pasting properties of corn starch as affected by hydrothermal modification by various methods. International Journal of Food Properties 2022, 25, 792-812.
50. Thakur, R.; Pristijono, P.; Golding, J.B.; Stathopoulos, C.; Scarlett, C.J.; Bowyer, M.C.; Singh, S.P.; Vuong, Q.V. Effect of starch physiology, gelatinization, and retrogradation on the attributes of rice starch‐ι‐carrageenan film. Starch-starke 2018, 70, 1700099.
51. Keirudin, A. A.; Zainuddin, N.; Yusof, N. A. Crosslinked Carboxymethyl Sago Starch/Citric Acid Hydrogel for Sorption of Pb2+, Cu2+, Ni2+ and Zn2+ from Aqueous Solution. Polymers 2020, 12, 2465.