1.0 Introduction
Despite the availability of malaria chemotherapy, malaria remains a life-threatening parasitic infectious disease in Sub-sahara Africa. It is caused by the protozoan parasites of Plasmodium species and is transmitted through bites of infected Anopheles mosquitoes of female species [1]. In humans, malaria is caused by five members of the Plasmodium species, including, falciparum, vivax, malariae, ovale, and knowlesi. The P. falciparum is, however, the main causative species of severe malaria and the most implicated in malaria-associated mortality [1, 2].
Malaria is particularly, prevalent in tropical and sub-tropical regions of the globe. According to the recent statistic, there were 212 million and 429, 000 malaria cases and death respectively in 2015, particularly in sub-Saharan Africa, where an estimated 90 % of the deaths occur in children under 5 years and expectant mothers [3]. Malaria is endemic all over the 36 states of the country with 97 % of the total population (approximately 173 million) being at risk of infection. In fact, Nigeria tops the list of malaria burdened countries worldwide. About 10 million cases and 300,000 deaths are annually documented [4, 5]. Besides, malaria accounts for 60 % of outpatient visits to health facilities, 30% of childhood death and 11 % maternal mortality [4, 6, 7].
The application of plant-based therapy for the management of malaria has long been practised with a high rate of ethnohistorical success [8]. Plant-based antimalarial has also been successfully applied to conventional therapy. For instance, the first antimalarial drug, quinine was isolated from the bark of medicinal plants known as Cinchona species (Rubiaceae). Another plant-based conventional therapy is Artemisinin, a fast-acting antiplasmodial drug isolated from Artemisia annua (Chinese sweet wormwood) [3] and also formed an important component of the Artemisinin-based combination therapies (ACTs). ACT has so far been explored as the first-line treatment option and the only available option for treatments of malaria resistance cases in countries [3]. However, cases of drug resistance to the combination therapy have been widely reported [3], suggesting the need for alternative fast-acting and safe therapeutic option. Hence the need to explore the therapeutic properties of medicinal plants
Psidium guajava, is a medicinal plant commonly known as guava (family Myrtaceae). All parts part, of Psidium guajava including the bark, fruit, leaves, and roots has a long reputation of therapeutic value in the management of several diseases including wound healing, diabetes, ulcers, hypertension, stomach ache, cholera, obesity, diarrhoea, trypanosomiasis and malaria infection [9, 10], and has been recognised as an important component of antimalarial polyherbal formulations by the traditional herbalist [9, 11]. However, scientific validation of its full antimalarial potency remains inadequate. Therefore, in this research, we evaluated the antimalarial activity of the crude extract and fractions of Psidium guajava leaf in Plasmodium berghei infected mice.
2.0 Materials and Methods
2.1. Collection of experimental parasites, plants and animals.
Fresh leaves of Psidium guajava was collected in July, 2015 from Minna, Niger State, Nigeria. The plant was identified by a taxonomist at the Botany Department of University of Nigeria Nsukka where a voucher number of UNH98 was deposited. Female Swiss albino mice, weighing between 22-28g were procured from National Veterinary Research Institute (NVRI), Vom, Plateau State of Nigeria. The experiment was conducted in strict compliance with the regulation of the Canadian Council on Animal Care (CCAC) guidelines on animal use protocol review [12]. The Plasmodium berghei (NK65) was obtained from the National Institute of Pharmaceutical Research and Development (NIPRD), Abuja, Nigeria. It was maintained in mice by serial blood passage.
2.2. Crude extract preparation and fractionation
The Psidium guajava were clean and pulverised into fine powder. Fifty (50) grams of the powdered sample was refluxly extracted in 400ml of 70% methanol for 2 h, as described previously [13]. The extracts were filtered and then concentrated to dryness under reduced pressure using a rotary evaporator. The crude extract was further fractionated into n-hexane, chloroform and ethyl acetate fraction and were concentrated under reduced pressure at 35oC using rotary evaporator
2.3 In vivo anti plasmodial study
Healthy mice were inoculated with P. berghei parasite as described previously [14]. The curative potential of plant extracts was evaluated by employing the method of [15]. Seventy-two hours later (day 3), following confirmation of parasitaemia, the infected mice were randomly assigned into 6 groups of 3 mice each and treated orally with the crude extract of P. guajava at doses of 100 (A), 300 (B) and 500 (C) mg/kg/day. The positive control groups (D& E) were treated with standard drugs, 5 mg/kg/day chloroquine and 50 mg/kg/day artesunate, respectively and 0.2 ml distilled water was administered to group F (negative control group). Group G were not infected but treated with 500 mg/kg body weight of the extract. All the treatments lasted for 5 days. Geimsa stained (10%) thin blood film was examined microscopically. The chloroform, ethyl acetate and aqueous fractions of Psidium guajava were also evaluated for antiplasmodial activity at doses of 50, 100 and 200 mg/kg/day. Average percentage parasitaemia and inhibition of parasite growth were calculated according to the following formulas [16]:
X100 |
% parasitemia = No of parasitized erythrocyte/ No of erythrocytes
% inhibition = % inhibition = A-B/A × 100
Where: A = Parasitaemia in negative control, B = Parasitaemia in test group
The body weight and packed cell volume of the mice were evaluated using a sensitive weighing balance and microhematocrit techniques while the mean survival time of the experimental mice was determined using the formula:
MST = Sum of survival time of all mice in a group/Total number of mice in that group
2.4 Phytochemical screening.
The crude extract of P. guajava was evaluated for phytochemical screening using standard procedures to identify the secondary metabolites as described by [17, 18].
2.5 Statistical analysis.
Results of the study were expressed as mean ± SEM. Data were analysed using Windows SPSS Version 19.0. One-way analysis of variance (ANOVA) coupled to Duncan (post-hoc test) was used to determine statistical significance. The result was considered significant at 95% confidence level and P-value < 0.05.
- Results
3.1. Antiplasmodial activity of crude extract of leaf of Psidium guajava.
The results showed a significant (p<0.05) antiplasmodial activity in all the extract-treated groups when compared with the negative control (infected but not treated) (Fig. 1). The antiplasmodial activity was in a dose dependent pattern with the maximum dose administered demonstrating the highest activity. The level of parasitaemia at 100, 300, and 500mg/kg was reduced by 56.19, 63.22 and 69.96% respectively as against 85.19 and 87.88% for the standard drugs chloroquine and artesunate respectively on day 8 relative to the negative control (Table 1). The variations in the levels of percentage Packed Cell Volume (%PCV) and body weight for the treated groups (infected but treated) before and after treatment was not significant (p > 0.05) whereas a slight increase in body weight and PCV was observed in not infected but treated (NIT) group (Fig. 2 and 3). Conversely, there was a significant (p < 0.05) decrease in body weight and PCV in negative control group. The survival time of the extract-treated groups was significantly (P < 0.05) prolonged when compared with the negative control group (Table 1).
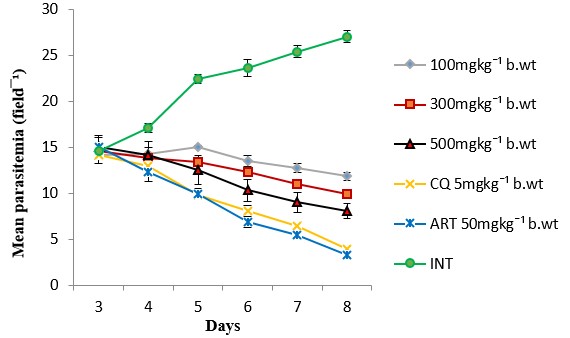
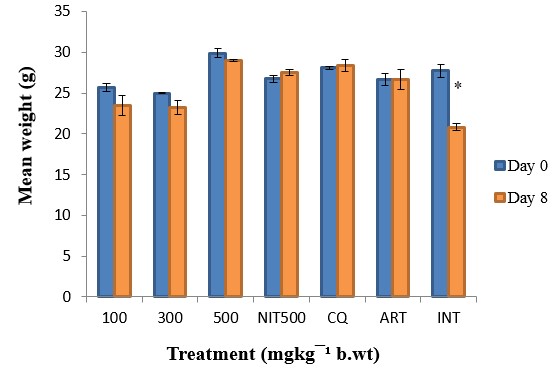
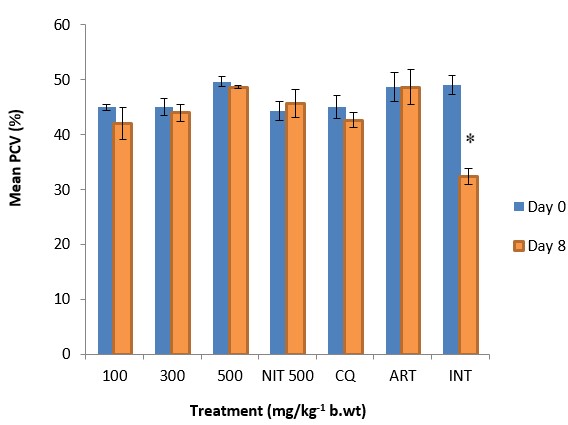
Figure 3: The effect of crude extract of Psidium guajava on PCV of P. berghei infected mice. Data are mean ± SEM; CQ = chloroquine (5mg/kg), ART = artesunate (50mg/kg), NIT = not infected but treated, INT = infected but not treated, * = significant difference between the %PCV before infection and after treatment
Table 1: Effect of aqueous methanolic extracts of Psidium guajava on the % inhibition of parasite growth and survival time of P. berghei infected mice
Dose (mg/kg) | Parasitaemia (Day 8) | % Inhibition | Mean Survival Time (Days) |
100 | 11.83±0.46 | 56.19 | 19.67±1.21 |
300 | 9.93±0.24 | 63.22 | 22.33±2.53 |
500 | 8.11±0.82 | 69.96 | 28.33±0.88 |
500 (NIT) | – | – | 30.00±0.00 |
CQ 5 | 4.00±0.23 | 85.19 | 27.33±1.76 |
ART 50 | 3.27±0.24 | 87.88 | 27.67±1.45 |
INT | 27.00±0.64 | 0 | 10.67±0.88 |
3.2. Antiplasmodial Activities of Fractions of Psidium guajava extract.
The antiplasmodial activity of the aqueous fraction of Psidium guajava extract at 200mg/kg body weight was highest among all the fraction-treated groups (Fig. 4 – 6) with 54.26% inhibition of parasite growth (Table 2). Meanwhile, inhibition of parasite growth was significantly less when compared with the crude extract (66.60%) and chloroquine (79.95%). Significant (p < 0.05) weight loss and reduction in PCV was observed in all the groups except in the groups treated with the crude extract, chloroquine and aqueous fraction (200mg/kg) (Fig. 7 and 8). The Survival time of fraction (200mg/kg) and crude extract-treated groups was significantly prolonged relative to the untreated control group (Table 2).
3.3. Phytochemical Screening.
Phytochemical screening of the crude extract revealed the presence of alkaloids, flavonoids, tannins, saponins, steroids, terpenoids and phenols.
Table 2: Effect of aqueous methanolic extracts of Psidium guajava on the % inhibition of parasite growth and survival time of P. berghei infected mice
Dose (mg/kg) | Parasitaemia (Day 8) | Inhibition (%) | Survival day |
C50 | 17.50±0.38 | 46.02 | 16.00±1.53 |
C100 | 17.25±0.38 | 46.79 | 18.67±2.40 |
C200 | 17.17±0.30 | 47.04 | 18.67±1.20 |
E50 | 20.50±0.29 | 36.77 | 15.67±1.33 |
E100 | 20.75±0.36 | 36.00 | 13.67±1.45 |
E200 | 18.70±0.00 | 42.17 | 15.00±1.16 |
A50 | 17.42±0.58 | 46.27 | 19.67±0.88 |
A100 | 15.83±0.74 | 51.17 | 19.67±1.76 |
A200 | 14.83±0.33 | 54.26 | 24.67±0.67 |
Cr50 | 10.83±0.65 | 66.60 | 27.33±1.76 |
CQ 5 | 6.50±0.63 | 79.95 | 28.00±1.16 |
INT | 32.42±0.36 | 0 | 8.33±0.67 |
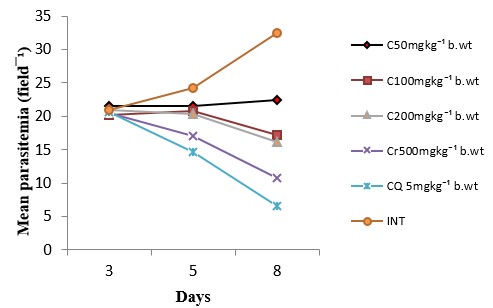
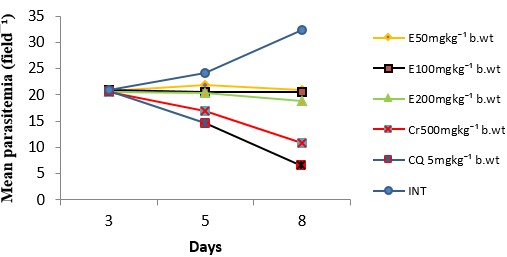
Figure 5: Antiplasmodial activity of ethyl acetate fraction of Psidium guajava . Data are mean ± SEM; n = 3, E = ethyl acetate fraction, Cr = crude extract, CQ = chloroquine, INT = infected but not treated (untreated control)
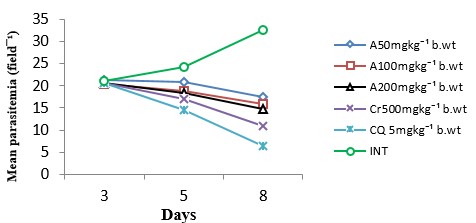
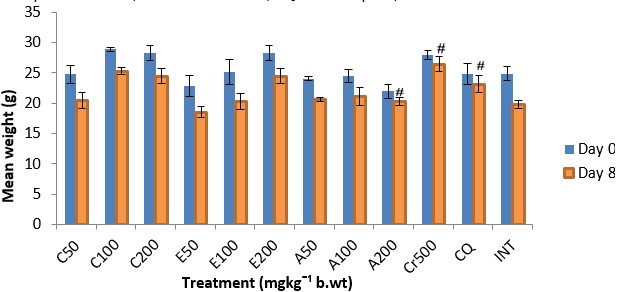
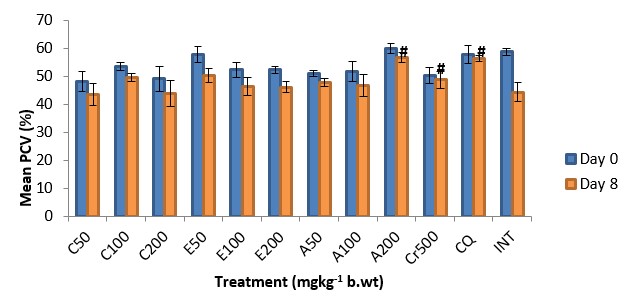
4.0 Discussion
Psidium guajava is used in the treatment of various ailments including malaria and has been reported to contain bioactive components [10]. In the present study, we evaluated the antimalarial potential of aqueous methanolic crude extract of Psidium guajava leaf and its fractions in P. berghei infection in mice. The rodent parasite, P. berghei, has been used extensively in the discovery and development of several orthodox antimalarial drugs corroborated by quinine, chloroquine, mefloquine and more recently artemisinin derivatives [8, 19, 20]. In addition, rodent parasite produces diseases similar to those of human plasmodial infection and is used in the prediction of treatment outcomes [21, 22]. This informed our choice for this parasite.
Determination of percentage inhibition of parasite growth is regarded as the most dependable parameter in antimalarial drug discovery [23]. A mean parasitemia level ≤ 90% to that of mock-treated control animals usually indicates that the test compound is active in standard screening studies [24, 25]. In the 5-day curative test, the aqueous methanolic crude extract of Psidium guajava leafdemonstrated asignificant (p < 0.05) antiplasmodial activity in a dose-dependent pattern indicating that the plant has antimalarial activity. The antimalarial activity of the aqueous crude leaf extract of the plant in early infection has also been reported by other investigators [24, 26].
Anaemia and body weight loss are common features of P. berghei infected mice [27, 28, 29]. Plants with antimalarial activity are expected to prevent body weight loss which is associated with loss of appetite in infected mice due to rising parasitaemia. Similarly, rodent malaria causes parasite-induced fall of PCV, which occurred approximately 48 h post-infection [29, 30]. Plasmodium berghei infected mice suffer from anaemia because of erythrocyte destruction, either by parasite multiplication or by spleen reticuloendothelial cell action as the presence of many abnormal erythrocytes stimulates the spleen to produce many phagocytes [8, 30]. All of these mechanisms account for malaria induced anaemia both in mice and men [31]. The crude extract of P. guajava significantly prevented body weight loss and reduction in PCV associated with increasing parasitemia level. The protective effect of P. guajava crude extract against body weight loss and PCV reduction infers its potency in ameliorating these common pathological features of malaria.
The mean survival time is important to evaluate the anti-malarial activity of plant extracts since it is associated with inhibition of parasite growth (Peters, 1975). The extension of survival period by the test compound beyond 12 days after killing the untreated control is an indication of being active [25, 32]. The mean survival time of mice treated with the extract was significantly longer than the untreated control mice with more than 12 days extension of life for mice in the 300 and 500mg/kg treated groups. This suggests that the extract suppressed P. berghei and reduced the overall pathologic effect of the parasite on the mice. However, neither the extracts nor the standard drug cured the infection. This could be due to the assertion that crude extracts tend to have better plasmodistatic than plasmodicidal effects as unpurified bioactive principles may require initial conversions which time lag allows for parasite proliferation [33, 34]. Furthermore, the active constituents might not be present in sufficient concentrations to effect rapid and complete clearance of parasites [35]. However, failure of the standard drug to completely clear the parasite could be due to recrudescence of P. berghei parasites after apparent cure. This may require more extensive investigation to ascertain what exactly is happening.
Alkaloids, flavonoids, phenols and terpenoids are known to possess antimalarial activity [36-39]. Alkaloids have been known to display antiplasmodial activities by blocking protein synthesis in Plasmodium falciparium [38, 40]. Flavonoids have been reported to chelate with nucleic acid base pairing of the parasite. Flavonoids are also reported to be involved in modulation of host immunity to tackle disease and inhibition of plasmodial enoyl-ACP reductase (FAB I enzyme), a key regulator of type II fatty synthases (FAS-II) in P. falciparum [41, 42]. Phenols and flavonoids are known for their anti-oxidant properties [37, 43]. It has been reported that antioxidant activity can inhibit heme polymerization as heme has to be oxidized before polymerization, and the unpolymerized heme is very toxic for malaria parasite [44-46]. Flavonoids may also bind parasite’s serine/threonine kinase with high affinity and affect its development [47]. Terpenoids have been implicated for their antiprotozoal and antimalarial activities in many pharmacological studies which are attributed to the endoperoxidation property of the phytochemical [39, 48]. The observed antimalarial activity in this study may be related to the presence of active compounds such as alkaloids, flavonoids, terpenoids and phenols in the extract.
The reduction in body weight gain and PCV is a simple and sensitive tool in toxicological study after exposure to toxic substance [29]. The crude extract administered to non-infected normal mice at 500mg/kg body weight per day for five days did not cause decrease in body weight and PCV of experimental mice. Rather body weight gain and increase in PCV were observed. All the experimental mice survived the 30 days observation period. Therefore, it can be inferred that the crude extract is safe for oral administration at the dose tested.
Among the fractions, the aqueous fraction was found to have significantly higher blood schizontocidal activity than the other fractions suggesting the possible localization of the active ingredients in this fraction. The aqueous fraction at the highest dose administered showed protective effect on body weight loss and PCV reduction. Furthermore, the aqueous fraction significantly (P < 0.05) prolonged the mean survival time of infected mice relative to other fractions and untreated control. The longest survival time of mice as a result of the administration of the highest dose of aqueous fraction suggests the presence of active secondary metabolites in sufficient concentration in that dose. Nevertheless, the crude extract demonstrated a significantly higher parasite inhibition than the aqueous fraction which indicates that there may be partial loss of activity upon fractionation of the crude extract. Similar observation was reported by other researchers [12, 33, 49]. This suggests that the active components of the crude extract may have synergistic effect when they coexist in the crude extract to exert a higher antimalarial activity. It is also possible that there are other components in the crude extract that exert a positive modulating effect on the bioactivity of the extract which may also have been lost on purification. However, in vivo antiplasmodial activity of plant extracts are classified as moderate, good and very good if an extract displays parasite inhibition ≥ 50% at respective doses of 500, 250 and 100mg/kg body weight per day [46, 50]. The aqueous fraction can be considered as having good antiplasmodial activity since it demonstrated > 50% growth inhibition when administered at a dose of 200mg/kg body weight per day for five days. Therefore, there is still the prospect of a better antilplasmodial activity upon further purification and isolation of the bioactive compound(s).
5.0 Conclusion
The results obtained from the present study have shown that the leaf extract of Psidium guajava as well as the fractions are endowed with antimalarial potentials. The crude extract can serve as a new source for the development of new and affordable antimalarial agent. Also, further purification of the most potent fraction can lead to isolation of novel antimalarial compounds.
Abbreviations: ANOVA; One-way analysis of variance, ART = artesunate, CCAC: Canadian Council on Animal Care, CQ = chloroquine, DMRT: Duncan’s Multiple Range Test, INT = infected but not treated, NIPRD; National Institute of Pharmaceutical Research and Development, NVRI: National Veterinary Research Institute, P. berghei=Plasmodium berghei, PCV: Packed Cell Volume, SEM: Standard error of mean, SPSS= Statistical package for social sciences
Ethics approval: The experiment was conducted in compliance with the internationally accepted principles for laboratory animal use and care as contained in the Canadian Council on Animal Care (CCAC) guidelines on animal use protocol review
Competing interests: The authors declared no conflict of interest exist
Funding: This work was funded, in part, by a TETFund Institutional Based Research grant to Emmanuel O. Ogbadoyi.
Authors’ contributions: All authors participate in research design. Author AUB conducted the research work and write the manuscript. Author EOO supervised the work and revised the manuscript while authors MA, and KAY co-supervised and revised the work. All authors read and approved the final manuscript.
Acknowledgements: This work was funded, in part, by a TETFund Institutional Based Research grant to Emmanuel O. Ogbadoyi.
References
[1] O. Okpe, N. Habila, J. Ikwebe, V. A. Upev, S. I. R. Okoduwa, and O. T. Isaac, “Antimalarial Potential of Carica papaya and Vernonia amygdalina in Mice Infected with Plasmodium berghei,” Journal of Tropical Medicine, 2016.
[2] D. Nigussie, T. Beyene, N. A. Shah, and S. Belew, “New Targets in Malaria Parasite Chemotherapy: A Review,” Malaria Control and Elimination, vol. 1, article 1, 2015.
[3] WHO (2016). World Health Organization Fact sheet on the World Malaria Report. http://www.who.int/malaria/media/world_malaria_report_2016/en/. Retrieved on 22nd May, 2017.
[4] WHO “World malaria report 2014,” Geneva: World Health Organization. http://www.who.int/malaria/media/world_malaria_report_2014/en/. Retrieved on 22nd May, 2017.
[5] Nigeria Federal Ministry of Health. “National Malaria Control Programme Strategic plan 2014–2020: a road map for malaria control in Nigeria, Abuja,” Yaliam Press Ltd, Federal Ministry of Health, 2014.
[6] USEN “Nigeria Malaria Fact Sheet,” Economic Section, United States Embassy in Nigeria, 2011. Retrieved 20th March, 2016.
[7] I. S. Dawak, M. A. Hesham, I. Ithoi, J. Ibrahim et al., “Is Nigeria winning the battle against malaria? Prevalence, risk factors and KAP assessment among Hausa communities in Kano State,” Malaria Journal, vol. 15, article 351, 2016.
[8] V. Somsak, N. Polwiang, and S. Chachiyo, “In vivo antimalarial activity of Annona muricata leaf extract in mice infected with Plasmodium berghei,” Journal of Pathogens, 2016.
[9] B. Joseph, and R. M. Priya, “Review on nutritional, medicinal and pharmacological Properties of guava (Psidium guajava linn.),” International Journal of Pharma and Bio Sciences, vol. 2, no. 1, 2011.
[10] S. M. Barbalho, F. M. V. Farinazzi-Machado, R. A. Goulart, A. C. S. Brunnati, A. M. M. B. Ottoboni, and C. C. T. Nicolau, “Psidium Guajava (Guava): A Plant of Multipurpose Medicinal Applications,” Medicinal and Aromatic Plants, vol. 1, article 4, 2012.
[11] O. A. Idowu, O. T. Soniran, O. Ajana, and D. O. Aworinde, (2010). Ethnobotanical survey of antimalarial plants used in Ogun State, Southwest Nigeria,” African Journal of Pharmacy and Pharmacology, vol. 4, no. 2, pp. 55-60, 2010.
[12] U. B. Ugwu, J. I. Okogun, A. Y. Kabiru, and E. O. Ogbadoyi, “Evaluation of Therapeutic Potentials of Stem Bark Extracts of Annona senegalensis in Experimental Trypanosoma brucei brucei Infection in Mice,” British Journal of Pharmacology and Toxicology, vol. 2. No. 2, 63-70, 2011.
[13] CCAC, “Guidelines on Animal use and Protocol review,” 1997. http://www.ccac.ca/Documents/Standards/Guidelines/Protocol_Review.pdf
[14] D. A. Fidock, P. J. Rosenthal, S. L. Croft, R. Brun, and S. Nwaka, “Antimalarial drug discovery: efficacy models for compound screening,” Nature Reviews and Drug Discovery, vol. 3, no. 6, 509-520, 2004.
[15] J. F. Ryley, and W. Peters, “The antimalarial activity of some quinoline esters,” Annal of Tropical Medicine and Parasitology, vol. 84, pp. 209-222, 1970.
[16] W. Peters, and H. Portus, and L. Robinson, “The four-day suppressive in vivo antimalarial test,” Annal of Tropical Medicine and Parasitology, vol. 69, pp. 155-171, 1975.
[17] A. Sofowora, “Medicinal plants and traditional medicine in Africa,” Spectrum Books, Ibadan, pp: 150, 1993.
[18] W. C. Evans, “Trease and Evans Pharmacognosy,” 13th Edition, Balliere Tindall, London, pp. 378-689, 1989.
[19] A. M. Thomas, A. M. van der Wel, A. W. Thomas, C. J. Janse, and A. P. Waters, “Transfection systems for animal models of malaria,” Parasitology Today, vol. 14, pp. 248-249, 1998.
[20] N. Nutham, S. Sakulmettatham, S. Klongthalay, P. Chutoam, and V. Somsak, “Protective effects of Tinospora crispa stem extract on renal damage and hemolysis during Plasmodium berghei infection in mice,” Journal of Pathogens and Current Science, vol. 85, pp. 1314-1329, 2015.
[21] M. C. English, C. Waruira, C. Lightowler, S. A. Murphy, G. Kirigha, and K. Marsh, “Hyponatreamia and dehydration in severe malaria,” Archives of Disease in Childhood, vol. 74, pp. 201-205, 1996.
[22] K. A. Kumar, S. Singn, and P. P. Babu, “Studies on the glycoprotein modification in erythrocyte membrane during experimental cerebral malaria,” Experimental Parasitology, vol. 114, pp. 173-179, 2006.
[23] F. Mojab, “Antimalarial natural products: a review,” Avicenna Journal of Phytomedicine, vol. 2, pp. 52-62, 2012.
[24] A. T. Arrey, F. Okalebo, A. S. Ayong, G. A. Agbor, and A. N. Guantai, “Anti-malarial activity of a polyherbal product (Nefang) during early and established Plasmodium infection in rodent models,” Malaria Journal, Vol. 13, p. 456, 2014.
[25] I. T. Peter, and V. K. Anatoli, “The Current Global Malaria Situation: Malaria Parasite Biology, Pathogenesis, and Protection,” Washington, DC, USA, ASM Press, 1998.
[26] C. Rajendran, M. Begam, D. Kumar et al., “Antiplasmodial activity of certain medicinal plants against chloroquine resistant Plasmodium berghei infected white albino BALB/c mice,” Journal of Parasitology and Diseases, vol. 38, no. 2, pp. 148-152, 2014.
[27] J. Langhorne, S. J. Quin, and L. A. Sanni, “Mouse models of blood-stage malaria infections: immune responses and cytokines involved in protection and pathology,” Chemistry and Immunology, vol. 80, pp. 204-228, 2002.
[28] L. Bantie, S. Assefa, T. Teklehaimanot, and E. Engidawork, “In vivo antimalarial activity of the crude leaf extract and solvent fractions of Croton macrostachyus Hocsht. (Euphorbiaceae) against Plasmodium berghei in mice,” Complementry and Alternative Medicine, vol. 14, article no. 79, 2014.
[29] A. Nardos, and E. Makonnen, “In vivo antiplasmodial activity and toxicological assessment of hydroethanolic crude extract of Ajuga remota,” Malaria Journal, vol. 16, article 25, 2017.
[30] M. Chinchilla, M. O. Guerrero, G. Abarca, M. Barrios, and O. Castro. “An in vivo model to study the anti-malaric capacity of plant extracts,” Revista de Biologia Tropical, vol. 46, no. 1, pp. 35-39, 1998.
[31] A. A. Lamikanra, M. Theron, T. W. A. Kooij, and D. J. Roberts, “Hemozoin (Malarial Pigment) Directly Promotes Apoptosis of Erythroid Precursors,” Public Library of Science one, vol. 4, no. 12, 2009.
[32] O. M. Nafiu, T. A. Abdulsalam, and M. A. Akanji, “Phytochemical Analysis and Antimalarial Activity of Aqueous Extract of Lecaniodiscus cupanioides Root,” Journal of Tropical Medicine, 2013.
[33] H. Noedl, C. Wongsrichanalai, and W. H. Wernsdorfer, “Malaria drug sensitivity testing: New assays, new perspectives,” Trends in Parasitology, vol. 19, pp. 175-181, 2003.
[34] A. Y. Kabiru, A. Abdulkadir, A. T. Gbodi, U. M. Bello, H. A. Makun, D. J. Amah, and E. O. Ogbadoyi, “Evaluation of Haematological Changes in Plasmodium-berghei-infected Mice Administered with Aqueous Extract of Phyllantus amarus,” Pakistan Journal of Biological sciences, vol. 16, no. 11, 510-516, 2013.
[35] A. Fidock, P. Rosenthal, S. Brun, and S. Nwaka, “Antimalarial drug discovery: Efficacy models for compound screening,” Natural Revolution and Drug Discovery, vol. 3, pp. 509-520, 2006.
[36] K. C. Lui, S. C. Yang, and M. F. Roberts, “Antimalarial activity of Artemisia annua flavonoids from whole plants and cell cultures,” Plants Cell, vol. 2, pp. 637-640, 1992.
[37] S. Saxena, N. Pant, D. Jain, and R. Bhakuni, “Antimalarial agents from plant sources,” Current Science, vol. 85, Article 9, 2003.
[38] J. E. Okokon, E. O. Etebong, J. A. Udobang, and J. Obot, “Antiplasmodial and antiulcer activities of Melanthera scadens,” Asian Pacific Journal of Tropical Medicine, vol. 2, pp. 16-20, 2012.
[39] S. A. Tadesse, and Z. B. Wubneh, “Antimalarial activity of Syzygium guineense during early and established Plasmodium infection in rodent models.” Complementary and Alternative Medicine, vol. 17, article 21, 2017,
[40] E. O. Ettebong, P. A. Nwafor, and J. E. Okokon, “In vivo antiplasmodial activities of ethanolic exract and fractions of Eleucine indica,” Asian Pacific Journal of Tropical Medicine, vol. 2, pp. 673-676, 2012.
[41] J. E. Okokon, B. S. Antia, B. A. Azare, and P. J. Okokon, “Antiplasmodial activity and cytotoxicity of ethanol extract of Zea mays root,” Avicenna Journal of Phytomedicine, vol. 7. No. 3, pp. 275-284, 2017.
[42] H. Kirmizibekmez, I. Calis, R. Perozzo, R. Brun, A. A. Donmez, and A. Linden, “Inhibiting activities of the secondary metabolites of Phlomis brunneogaleata against parasitic protozoa and plasmodial enoyl-ACP reductase, a crucial enzyme in fatty acid biosynthesis,” Planta Medica, vol. 70, article 711, 2004.
[43] V. Somsak, P. Borkaew, C. Klubsri, K. Dondee, P. Bootprom, and B. Saiphet, “Antimalarial Properties of Aqueous Crude Extracts of Gynostemma pentaphyllum and Moringa oleifera Leaves in Combination with Artesunate in Plasmodium berghei-Infected Mice,” Journal of Tropical Medicine, 2016.
[44] P. A. Berman, and P. A. Adams “Artemisinin enhances hemecatalysed oxidation of lipid membranes,” Free Radical Biology and Medicine, vol. 22, no. 7, pp. 1283-1288, 1997.
[45] B. Mengiste, E. Makonnen, and K. Urga, “In-vivo antimalarial activity of Dodonaea angustifolia seed extracts against Plasmodium Berghei in mice model,” Momona Ethiopian Journal of Science, vol. 4, no. 1, 47-63, 2012.
[46] S. Fentahun, E. Makonnen, T. Awas, and M. Giday, “In vivo antimalarial activity of crude extracts and solvent fractions of leaves of Strychnos mitis in Plasmodium berghei infected mice,” Complementary and Alternative Medicine, vol. 17, pp. 13-19, 2017.
[47] J. F. Ferreira, D. L. Luthria, T. Sasaki, and A. Heyerick, “Flavonoids from Artemisia annua L. as antioxidants and their potential synergism with artemisinin against malaria and cancer. Molecules,” vol. 15, pp. 3135-3170, 2010.
[48] P. Ghildyal, T. Gronhuag, A. Rusten, and M. Skogsurd. “Chemical composition and immunological activities of polysaccharides isolated from the Malian medicinal plant Syzygium guineense,” Journal of Pharmacognosy and Phytotherapy, vol. 2, pp. 76–85, 2010.
[49] V. C. Ukwe, E. A. Epueke, O. I. Ekwunife, T. C. Okoye, G. C. Akudor, and C. M. Ubaka, “Antimalarial activity of aqueous extract and fractions of leaves of Ageratum conyzoides in mice infected with Plasmodium berghei,” International Journal of Pharmaceutical Sciences, vol. 2, pp. 33-38, 2010. [50] E. Deharo, G. Bourdy, C. Quenevo, V. Munoz, G. Ruiz, and M. A. Sauvain, “Search for natural bioactive compounds in Bolivia through a multidisciplinary approach: Part V. Evaluation of the antimalarial activity of plants used by the Tecana Indians,” Journal of Ethnopharmacology, vol. 77, no 1, pp. 91-98, 2001